Ocean Thermal Energy Conversion (OTEC): A Complete Introduction
— user
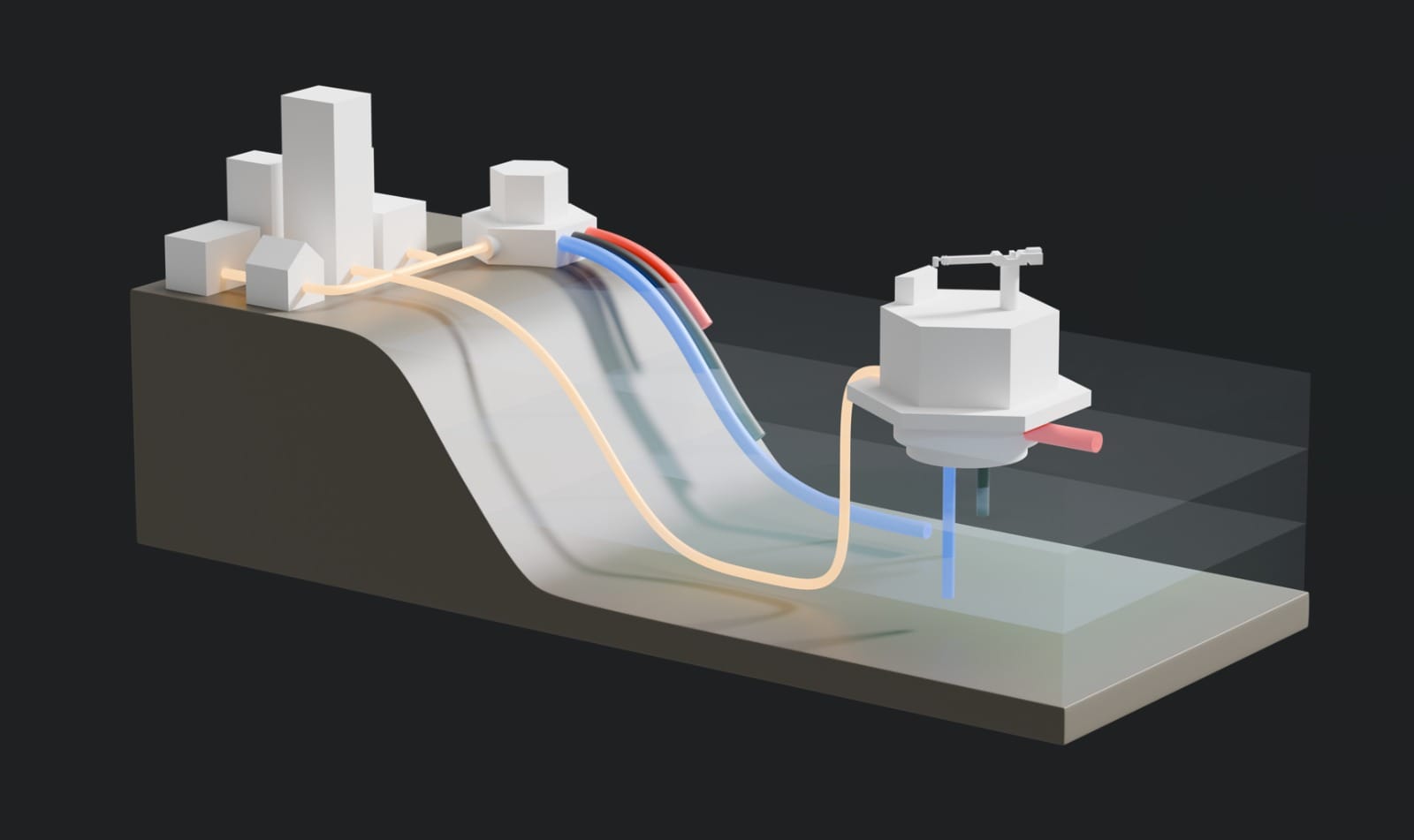
1. Introduction: Why the Ocean is Key to Our Energy Future
As the global energy sector pivots toward renewable solutions, most attention is focused on well-established technologies like solar, wind, and hydropower. However, hidden in the depths of our oceans lies an immense and underutilized resource—Ocean Thermal Energy Conversion (OTEC). OTEC exploits the temperature differential between warm surface waters and the colder waters of the deep ocean to generate clean, renewable electricity, offering an alternative capable of delivering consistent, base-load power in tropical regions.
While solar and wind technologies are subject to natural variability, OTEC’s ability to generate energy continuously—24 hours a day, 365 days a year—makes it an attractive yet often overlooked contributor to the future renewable energy mix. Despite this promise, OTEC remains largely undeveloped, restrained by technological and financial challenges. With recent advancements and a growing focus on sustainable energy, OTEC is poised to take on a more prominent role in the global energy landscape.
2. How OTEC Works: Tapping into the Ocean’s Thermal Gradient
OTEC operates on a simple but powerful principle: leveraging the ocean’s vertical thermal gradient to generate electricity. In tropical regions, where surface water temperatures are significantly higher than the cold, deep ocean waters, this temperature differential can drive a heat engine that produces continuous power.
The Science Behind OTEC: Utilizing Temperature Differentials
In tropical oceans, surface water temperatures often exceed 25°C (77°F), while water just a kilometer below the surface can drop to a frigid 5°C (41°F). OTEC systems capitalize on this gradient. Warm surface water is used to vaporize a working fluid—often ammonia—which drives a turbine connected to an electricity generator. Cold deep water is then pumped up to condense the vapor back into liquid form, allowing the cycle to repeat endlessly.
Closed-Cycle vs. Open-Cycle OTEC Systems
OTEC systems are categorized as either closed-cycle or open-cycle.
In closed-cycle systems, a working fluid such as ammonia circulates in a closed loop, vaporizing under heat from the warm surface water and then condensing as it is cooled by deep water. This process allows for efficient, large-scale power generation.
In open-cycle systems, seawater itself is the working fluid. Warm surface water is flash-evaporated in a low-pressure environment, creating steam that drives the turbine. The steam is then condensed by cold deep water, yielding both electricity and fresh desalinated water as a by-product. Each system has its advantages, with closed-cycle being more scalable and open-cycle producing valuable fresh water.
The Power of the Thermocline: 24/7 Energy Production
OTEC distinguishes itself from other renewables by offering continuous, uninterrupted energy production. The ocean’s thermal gradient remains stable year-round, especially in tropical regions, which allows OTEC to generate electricity around the clock. This 24/7 capability makes OTEC a reliable source of base-load power, capable of supplying energy consistently, unlike intermittent sources such as solar and wind.
Comparing OTEC to Other Renewable Energy Sources
While solar and wind technologies are integral to the renewable energy mix, they are inherently intermittent—solar power is unavailable at night, and wind energy is dependent on weather patterns. Hydropower, although reliable, is geographically limited. OTEC, on the other hand, offers continuous energy generation and can be deployed in many tropical regions, providing a stable complement to these other forms of renewable energy.
3. OTEC’s Practical Benefits: More Than Just Electricity
While OTEC’s primary function is power generation, its ancillary benefits make it particularly appealing for tropical and island regions. Beyond electricity, OTEC offers solutions for water scarcity, cooling, and food production.
Base-Load Energy for Tropical Regions
Tropical regions, particularly island nations, often face significant challenges in securing reliable and affordable energy. Many of these regions are dependent on imported fossil fuels, which are subject to volatile pricing and supply chain disruptions. OTEC offers a dependable, renewable source of electricity that reduces reliance on external energy sources, enhancing energy security and fostering sustainability.
Freshwater Production: Addressing Global Water Scarcity
One of OTEC’s most significant benefits, especially for regions facing freshwater scarcity, is its ability to produce fresh water as a by-product in open-cycle systems. By condensing steam produced from evaporated seawater, OTEC systems can generate potable water in addition to electricity, offering a sustainable solution to water scarcity—a growing concern for many island and coastal communities.
Seawater Air Conditioning (SWAC): Sustainable Cooling Solutions
OTEC systems can also provide energy-efficient cooling through Seawater Air Conditioning (SWAC). By using the cold water from the ocean depths, SWAC systems can cool buildings with a fraction of the energy typically required for conventional air conditioning systems. This technology is particularly valuable in tropical regions where air conditioning represents a major portion of energy consumption. SWAC can reduce energy demand by up to 90%, offering a highly efficient, low-emission cooling alternative.
Additional By-Products: Aquaculture and Agriculture
The cold, nutrient-rich water pumped from the ocean’s depths also has important implications for food production. This water can support aquaculture, enabling the cultivation of fish and other marine life, and can be used for agricultural irrigation. Furthermore, nutrient-rich deep water can enhance plant growth, offering a sustainable method to support agriculture in regions where fresh water and nutrients are limited.
4. OTEC in Action: Successful Case Studies
Several OTEC projects around the world have successfully demonstrated the feasibility and value of this technology. These projects offer insights into the potential for scaling OTEC and overcoming the challenges that have historically limited its development.
The Makai Ocean Engineering Plant in Hawaii
Hawaii is home to one of the most advanced OTEC systems in operation today—the Makai Ocean Engineering Plant. Since its inception in 2015, the plant has demonstrated that OTEC can be both a reliable and scalable solution, generating electricity and connecting to the local grid. The Makai plant serves as a critical testbed for future larger-scale deployments and provides a clear example of OTEC’s viability in island settings.
Japan’s Kumejima Island: A Model for Sustainable Development
On Kumejima Island, Japan, OTEC is part of a broader vision for sustainable island development. The island’s OTEC plant not only generates power but also provides cold water for aquaculture, supporting local fisheries, and serves as a resource for district cooling. The success of Kumejima’s OTEC system offers a compelling case for how small island developing states (SIDS) can leverage OTEC for energy independence and economic development.
South Korea’s K-OTEC1000 Floating Plant
South Korea’s K-OTEC1000, a floating OTEC plant, highlights the potential for offshore deployment. Floating plants are particularly valuable in regions where the necessary thermal gradient exists far from the coastline, making land-based systems impractical. The K-OTEC1000 plant demonstrates how floating platforms can expand OTEC’s reach and unlock its potential in offshore environments.
5. Overcoming Barriers: Scaling OTEC for Global Impact
Despite its promise, OTEC faces significant technological and financial barriers to widespread adoption. Addressing these challenges is essential to unlocking OTEC’s full potential.
The Cost Challenge: Addressing High CAPEX and LCOE
One of the primary obstacles to OTEC’s broader adoption is its high capital expenditure (CAPEX) and levelized cost of energy (LCOE). The infrastructure required for OTEC—particularly deep-water intake pipes—represents a significant upfront cost. However, as with many other renewable technologies, costs are expected to decrease over time as economies of scale are realized and technological advancements improve system efficiency.
Technology Readiness Levels (TRL): Current Status of OTEC Systems
OTEC technology is still maturing, with most projects in the pilot or demonstration phase. However, significant progress has been made in key areas such as cold water pipes (CWPs) and floating platforms, bringing OTEC closer to full commercial viability. Continued investment in research and development is critical to moving OTEC from its current state to large-scale deployment.
Engineering Breakthroughs: Cold Water Pipes and Floating Platforms
One of the most significant engineering challenges for OTEC is the construction and maintenance of durable, cost-effective cold water pipes, which must reach depths of 1,000 meters or more to access cold ocean water. Recent advancements in materials and construction techniques, many borrowed from the offshore oil and gas industry, have made these pipes more reliable and affordable, bringing large-scale floating OTEC plants within reach.
Policy and Regulatory Gaps: The Role of Government
For OTEC to achieve its full potential, supportive government policies are essential. Feed-in tariffs, tax incentives, and funding for demonstration projects can help mitigate the high upfront costs associated with OTEC systems and encourage private investment. Additionally, clear regulatory frameworks for offshore development are necessary to streamline project approval and reduce risk for developers.
6. Environmental and Societal Impact of OTEC
Beyond its energy and economic benefits, OTEC offers substantial environmental and societal advantages, making it a key player in the global transition to sustainable energy.
Minimal Environmental Footprint: No Fuel, No Emissions
Unlike fossil fuel-based power plants, OTEC produces no direct emissions and does not rely on fuel combustion. This makes OTEC one of the cleanest energy generation methods available. Its operational footprint is also relatively small, especially for floating plants, minimizing disruption to marine ecosystems.
Addressing Concerns: Deep-Water Upwelling and Ecosystem Impacts
Some environmental concerns have been raised about the effects of pumping nutrient-rich deep water to the surface, particularly the potential for algal blooms and ecosystem disruption. However, with proper management and release strategies, these risks can be minimized. In fact, controlled upwelling could enhance local marine productivity, contributing to sustainable fisheries and food security.
OTEC’s Role in Decarbonization: Long-Term Potential for Carbon Removal
OTEC’s capacity to operate as a carbon-neutral energy source positions it as a vital tool in global decarbonization efforts. Additionally, by stimulating marine productivity through upwelling, OTEC could contribute to carbon dioxide removal (CDR) from the atmosphere, enhancing its long-term environmental benefits.
How OTEC Can Benefit Small Island Developing States (SIDS)
For small island nations, OTEC offers not only energy independence but also economic and environmental resilience. By providing a stable source of renewable energy and freshwater, OTEC can reduce reliance on imported fuels and address critical issues such as water scarcity and food security, making it a transformative technology for SIDS.
7. Future Prospects: Scaling OTEC for Global Deployment
OTEC has the potential to play a pivotal role in the global energy transition. However, achieving this vision will require sustained investment, technological innovation, and policy support.
Moving from 2.5 MW to 100 MW and Beyond
Current OTEC projects are small in scale, but the technology is poised for expansion. Moving from 2.5 MW demonstration plants to 100 MW commercial-scale systems will be a critical step in proving OTEC’s viability as a global energy solution. Floating OTEC platforms, in particular, offer tremendous potential for scaling up in regions where onshore deployment is impractical.
Combining OTEC with Hydrogen and Ammonia Production
One of the most promising future applications of OTEC is its integration with hydrogen and ammonia production. By using OTEC-generated electricity to produce hydrogen or ammonia offshore, OTEC plants could serve as hubs for clean fuel production, contributing to global decarbonization efforts and providing an alternative to traditional fossil fuels.
The Role of Floating OTEC Plants in the Global Energy Mix
Floating OTEC plants offer a flexible and scalable solution for regions where deep water is not easily accessible from land. These platforms can be deployed in international waters, tapping into the vast thermal gradients of the open ocean. As the technology matures, floating OTEC systems could become a significant contributor to the global renewable energy mix.
Partnerships and Investments: What’s Needed to Make OTEC Mainstream
To bring OTEC into the mainstream, collaboration between governments, industry, and academia is essential. Public-private partnerships, combined with targeted investments in research, development, and deployment, will accelerate OTEC’s growth. Additionally, international cooperation will be crucial in establishing regulatory frameworks and sharing best practices to ensure the safe and efficient deployment of OTEC systems worldwide.
OTEC’s Place in the Renewable Energy Revolution
OTEC offers a unique combination of benefits—continuous power generation, fresh water production, and environmental sustainability—that make it an essential player in the renewable energy landscape. While challenges remain, technological advancements and policy support can unlock OTEC’s full potential, positioning it as a key contributor to the global energy transition. The time has come for governments, investors, and the public to recognize the value of OTEC and support its development as a cornerstone of our sustainable future.